Chronic Effects of Fusarium Mycotoxins in Rations with or without Increased Concentrate Proportion on the Insulin Sensitivity in Lactating Dairy Cows
Abstract
: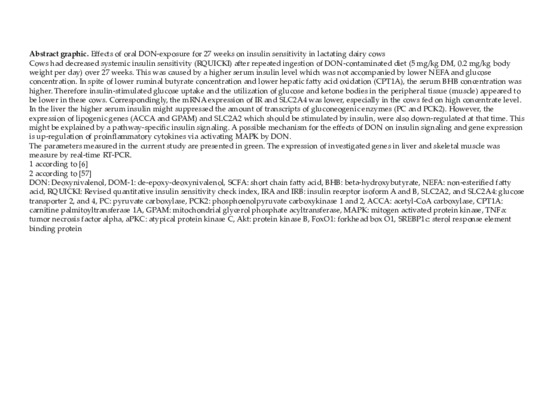
Graphical Abstract
1. Introduction
2. Results
2.1. Dry Matter Intake, Body Weight, Milk Production Performance, and Mycotoxin Intake
2.2. Blood Metabolites and Insulin Sensitivity
2.3. Muscular mRNA Expression of Glucose Transporters and Insulin Receptors
2.4. Hepatic mRNA Expression of Enzymes Involved in Gluconeogenesis and Oxidation and Esterification of Fatty Acid
3. Discussion
3.1. Study Design, Dose of DON, and Effect of Co-Contamination by Zearalenone
3.2. Blood Analysis and RQUICKI
3.3. Muscular and Hepatic mRNA Expression of Glucose Transporter 1 (SLC2A1)
3.4. Muscular mRNA Expression of Glucose Transporter (SLC2A4) and Insulin Receptor (IR)
3.5. Hepatic Insulin Receptor Expression
3.6. Hepatic mRNA Expression of SLC2A2 and Key Enzymes in Gluconeogenesis and Fatty Acid Metabolism
3.6.1. Lower Expression of Hepatic Enzymes Involved in Gluconeogenesis and Fatty Acid Metabolism in MYC Groups
3.6.2. Possible Explanation for the Suppression of Hepatic SLC2A2 Expression by DON
3.6.3. Lower Lipogenic Gene Expression in MYC Might Be Due to Reduced Hepatic Insulin Sensitivity
3.6.4. Lower mRNA Expression of PC and CPT1A in MYC Might Be Due to Higher Serum Insulin Level
3.6.5. Changes in Hepatic Insulin Sensitivity May Be Related to Hepatic Gene Expression
3.7. Possible Mechanisms of DON Effects on Insulin Sensitivity
4. Conclusions
5. Materials and Methods
5.1. Animals and Feeding
5.2. Collection of Blood and Tissue Samples
5.3. Analyses of Blood Samples
5.4. Analyses of Tissue Samples
5.4.1. RNA Isolation
5.4.2. Reverse Transcription
5.4.3. Real-Time qPCR Assay
5.5. Statistical Analysis
Supplementary Materials
Author Contributions
Funding
Acknowledgments
Conflicts of Interest
Abbreviations
ACCA | Acetyl-CoA carboxylase |
ACSL1 | Acyl-CoA synthetase long-chain family member 1 |
BDH2 | 3-hydroxybutyrate dehydrogenase, type 2 |
BHB | Beta-hydroxybutyrate |
BLAST | Basic Local Alignment Search Tool |
BW | Body weight |
CON | Control group |
CON30 or CON60 | Control group with 30% or 60% concentrate proportion in the diet |
CPT1A | Carnitine palmitoyltransferase 1A |
DGAT1 | Diacylglycerol O-acyltransferase homolog 1 |
DIM | Days in milk |
DM | Dry matter |
DMI | Dry matter intake |
DON | Deoxynivalenol |
DOM-1 | De-epoxy-deoxynivalenol |
FASN | Fatty acid synthase |
FBP1 | Fructose-1,6-bisphosphatase 1 |
FoxO1 | Forkhead box O1 |
G6Pase | Glucose-6-phosphatase |
GPAM | Mitochondrial glycerol phosphate acyltransferase |
GUSB | β-glucuronidase |
HMGL | 3-hydroxymethyl-3-methylglutaryl-CoA lyase |
HMGS2 | 3-hydroxy-3-methylglutaryl-coa synthase 2 |
IL | Interleukin |
IR | Insulin receptor |
IRA and IRB | Insulin receptor isoform A and B |
LSM | Least square means |
MRPS15 | Mitochondrial ribosomal protein S15 |
MYC | Mycotoxin group |
MYC30 or MYC60 | Mycotoxin group with 30% or 60% concentrate proportion in the diet |
NEFA | Non-esterified fatty acid |
PC | Pyruvate carboxylase |
PCCA | Propionyl-CoA carboxylase, alpha polypeptide |
PCK1 and PCK2 | Phosphoenolpyruvate carboxykinase 1 and 2 |
Real-time qPCR | Real-time quantitative polymerase chain reaction |
RQUICKI | Revised quantitative insulin sensitivity check index |
RPS9 | Ribosomal protein S9 |
SCFA | Short chain fatty acid |
SLC2A1, SLC2A2, and SLC2A4 | Glucose transporter 1, 2, and 4 |
SREBP1c | Sterol response element binding protein |
TNFa | Tumor necrosis factor alpha |
UXT | Ubiquitously-expressed transcript |
ZEN | Zearalenone |
References
- Döll, S.; Dänicke, S. The Fusarium toxins deoxynivalenol (DON) and zearalenone (ZON) in animal feeding. Prev. Vet. Med. 2011, 102, 132–145. [Google Scholar] [CrossRef] [PubMed]
- Pestka, J.J.; Smolinski, A.T. Deoxynivalenol: Toxicology and potential effects on humans. J. Toxicol. Environ. Health B Crit. Rev. 2005, 8, 39–69. [Google Scholar] [CrossRef] [PubMed]
- Dänicke, S.; Matthaus, K.; Lebzien, P.; Valenta, H.; Stemme, K.; Ueberschar, K.-H.; Razzazi-Fazeli, E.; Bohm, J.; Flachowsky, G. Effects of Fusarium toxin-contaminated wheat grain on nutrient turnover, microbial protein synthesis and metabolism of deoxynivalenol and zearalenone in the rumen of dairy cows. J. Anim. Physiol. Anim. Nutr. 2005, 89, 303–315. [Google Scholar] [CrossRef] [PubMed]
- Dänicke, S.; Brezina, U. Kinetics and metabolism of the Fusarium toxin deoxynivalenol in farm animals: Consequences for diagnosis of exposure and intoxication and carry over. Food Chem. Toxicol. 2013, 60, 58–75. [Google Scholar] [CrossRef] [PubMed]
- Kleen, J.L.; Hooijer, G.A.; Rehage, J.; Noordhuizen, J.P.T.M. Subacute ruminal acidosis (SARA): A review. J. Vet. Med. A Physiol. Pathol. Clin. Med. 2003, 50, 406–414. [Google Scholar] [CrossRef] [PubMed]
- Keese, C.; Meyer, U.; Rehage, J.; Spilke, J.; Boguhn, J.; Breves, G.; Dänicke, S. Ruminal fermentation patterns and parameters of the acid base metabolism in the urine as influenced by the proportion of concentrate in the ration of dairy cows with and without Fusarium toxin-contaminated triticale. Arch. Anim. Nutr. 2008, 62, 287–302. [Google Scholar] [CrossRef] [PubMed]
- Fink-Gremmels, J. The role of mycotoxins in the health and performance of dairy cows. Vet. J. Lond. Engl. 1997 2008, 176, 84–92. [Google Scholar] [CrossRef] [PubMed]
- Seeling, K.; Lebzien, P.; Dänicke, S.; Spilke, J.; Südekum, K.-H.; Flachowsky, G. Effects of level of feed intake and Fusarium toxin-contaminated wheat on rumen fermentation as well as on blood and milk parameters in cows. J. Anim. Physiol. Anim. Nutr. 2006, 90, 103–115. [Google Scholar] [CrossRef] [PubMed]
- Kinoshita, A.; Keese, C.; Beineke, A.; Meyer, U.; Starke, A.; Sauerwein, H.; Dänicke, S.; Rehage, J. Effects of Fusarium mycotoxins in rations with different concentrate proportions on serum haptoglobin and hepatocellular integrity in lactating dairy cows. J. Anim. Physiol. Anim. Nutr. 2015, 99, 887–892. [Google Scholar] [CrossRef] [PubMed]
- Takayama, H.; Shimada, N.; Mikami, O.; Murata, H. Suppressive effect of deoxynivalenol, a Fusarium mycotoxin, on bovine and porcine neutrophil chemiluminescence: An in vitro study. J. Vet. Med. Sci. 2005, 67, 531–533. [Google Scholar] [CrossRef] [PubMed]
- Wada, K.; Nabae, A.; Higuchi, H.; Nagahata, H. Effects of mycotoxins on chemiluminescent response and cytokine mRNA expression of bovine neutrophils. J. Vet. Med. Sci. 2010, 72, 1507–1511. [Google Scholar] [CrossRef] [PubMed]
- Dänicke, S.; Keese, C.; Goyarts, T.; Döll, S. Effects of deoxynivalenol (DON) and related compounds on bovine peripheral blood mononuclear cells (PBMC) in vitro and in vivo. Mycotoxin Res. 2011, 27, 49–55. [Google Scholar] [CrossRef] [PubMed]
- Korosteleva, S.N.; Smith, T.K.; Boermans, H.J. Effects of feedborne Fusarium mycotoxins on the performance, metabolism, and immunity of dairy cows. J. Dairy Sci. 2007, 90, 3867–3873. [Google Scholar] [CrossRef] [PubMed]
- Korosteleva, S.N.; Smith, T.K.; Boermans, H.J. Effects of feed naturally contaminated with Fusarium mycotoxins on metabolism and immunity of dairy cows. J. Dairy Sci. 2009, 92, 1585–1593. [Google Scholar] [CrossRef] [PubMed]
- Pestka, J.J. Deoxynivalenol: Mechanisms of action, human exposure, and toxicological relevance. Arch. Toxicol. 2010, 84, 663–679. [Google Scholar] [CrossRef] [PubMed]
- Maresca, M. From the Gut to the Brain: Journey and Pathophysiological Effects of the Food-Associated Trichothecene Mycotoxin Deoxynivalenol. Toxins 2013, 5, 784–820. [Google Scholar] [CrossRef] [PubMed]
- Pestka, J.J.; Zhou, H.-R.; Moon, Y.; Chung, Y.J. Cellular and molecular mechanisms for immune modulation by deoxynivalenol and other trichothecenes: Unraveling a paradox. Toxicol. Lett. 2004, 153, 61–73. [Google Scholar] [CrossRef] [PubMed]
- Riley, R.T.; Pestka, J. Mycotoxins: Metabolism, mechanisms and biochemical markers. In The Mycotoxin Blue Book; Diaz, D.E., Ed.; Nottingham University Press: Nottingham, UK, 2005; pp. 279–291. [Google Scholar]
- Holtenius, P.; Holtenius, K. A model to estimate insulin sensitivity in dairy cows. Acta Vet. Scand. 2007, 49, 29. [Google Scholar] [CrossRef] [PubMed]
- De Koster, J.D.; Opsomer, G. Insulin resistance in dairy cows. Vet. Clin. N. Am. Food Anim. Pract. 2013, 29, 299–322. [Google Scholar] [CrossRef] [PubMed]
- Guyot, H.; Detilleux, J.; Lebreton, P.; Garnier, C.; Bonvoisin, M.; Rollin, F.; Sandersen, C. Comparison of various indices of energy metabolism in recumbent and healthy dairy cows. PLoS ONE 2017, 12, e0169716. [Google Scholar] [CrossRef] [PubMed]
- Hayirli, A. The role of exogenous insulin in the complex of hepatic lipidosis and ketosis associated with insulin resistance phenomenon in postpartum dairy cattle. Vet. Res. Commun. 2006, 30, 749–774. [Google Scholar] [CrossRef] [PubMed]
- Keese, C.; Meyer, U.; Rehage, J.; Spilke, J.; Boguhn, J.; Breves, G.; Dänicke, S. On the effects of the concentrate proportion of dairy cow rations in the presence and absence of a fusarium toxin-contaminated triticale on cow performance. Arch. Anim. Nutr. 2008, 62, 241–262. [Google Scholar] [CrossRef] [PubMed]
- European Commission. Commission Recommendation of 17 August 2006 on the presence of deoxynivalenol, zearalenone, ochratoxin A, T-2 and HT-2 and fumonisins in products intended for animal feeding (2006/576/EC). Off. J. Eur. Union 2006, L 229, 7–9. [Google Scholar]
- Trenholm, H.L.; Thompson, B.K.; Hartin, K.E.; Greenhalgh, R.; McAllister, A.J. Ingestion of vomitoxin (deoxynivalenol)-contaminated wheat by nonlactating dairy cows. J. Dairy Sci. 1985, 68, 1000–1005. [Google Scholar] [CrossRef]
- Côté, L.M.; Dahlem, A.M.; Yoshizawa, T.; Swanson, S.P.; Buck, W.B. Excretion of deoxynivalenol and its metabolite in milk, urine, and feces of lactating dairy cows. J. Dairy Sci. 1986, 69, 2416–2423. [Google Scholar] [CrossRef]
- Charmley, E.; Trenholm, H.L.; Thompson, B.K.; Vudathala, D.; Nicholson, J.W.; Prelusky, D.B.; Charmley, L.L. Influence of level of deoxynivalenol in the diet of dairy cows on feed intake, milk production, and its composition. J. Dairy Sci. 1993, 76, 3580–3587. [Google Scholar] [CrossRef]
- Hochsteiner, W.; Schuh, M.; Luger, K.; Baumgartner, W. Effect of mycotoxin contaminated feed on production parameters of dairy cows. Berl. Münch. Tierärztl. Wochenschr. 2000, 113, 14–21. [Google Scholar] [PubMed]
- Mineo, H.; Hashizume, Y.; Hanaki, Y.; Murata, K.; Maeda, H.; Onaga, T.; Kato, S.; Yanaihara, N. Chemical specificity of short-chain fatty acids in stimulating insulin and glucagon secretion in sheep. Am. J. Physiol. 1994, 267, E234–E241. [Google Scholar] [CrossRef] [PubMed]
- Mueckler, M. Facilitative glucose transporters. Eur. J. Biochem. FEBS 1994, 219, 713–725. [Google Scholar] [CrossRef]
- Abe, H.; Kawakit, Y.; Hodate, K.; Saito, M. Postnatal development of glucose transporter proteins in bovine skeletal muscle and adipose tissue. J. Vet. Med. Sci. 2001, 63, 1071–1075. [Google Scholar] [CrossRef] [PubMed]
- Dühlmeier, R.; Voigt, J.; Breves, G.; Sallmann, H.P. Contents of the glucose transporters GLUT1 and GLUT4 in oxidative and glycolytic muscles of goat kids and adult goats. DTW Dtsch. Tierärztl. Wochenschr. 2005, 112, 408–413. [Google Scholar] [PubMed]
- Dühlmeier, R.; Sammet, K.; Widdel, A.; von Engelhardt, W.; Wernery, U.; Kinne, J.; Sallmann, H.-P. Distribution patterns of the glucose transporters GLUT4 and GLUT1 in skeletal muscles of rats (Rattus norvegicus), pigs (Sus scrofa), cows (Bos taurus), adult goats, goat kids (Capra hircus), and camels (Camelus dromedarius). Comp. Biochem. Physiol. A Mol. Integr. Physiol. 2007, 146, 274–282. [Google Scholar] [CrossRef] [PubMed]
- Im, S.-S.; Kwon, S.-K.; Kim, T.-H.; Kim, H.-I.; Ahn, Y.-H. Regulation of glucose transporter type 4 isoform gene expression in muscle and adipocytes. IUBMB Life 2007, 59, 134–145. [Google Scholar] [CrossRef] [PubMed]
- Bonnet, M.; Faulconnier, Y.; Hocquette, J.-F.; Bocquier, F.; Leroux, C.; Martin, P.; Chilliard, Y. Nutritional status induces divergent variations of GLUT4 protein content, but not lipoprotein lipase activity, between adipose tissues and muscles in adult cattle. Br. J. Nutr. 2004, 92, 617–625. [Google Scholar] [CrossRef] [PubMed]
- Graugnard, D.E.; Piantoni, P.; Bionaz, M.; Berger, L.L.; Faulkner, D.B.; Loor, J.J. Adipogenic and energy metabolism gene networks in longissimus lumborum during rapid post-weaning growth in Angus and Angus × Simmental cattle fed high-starch or low-starch diets. BMC Genom. 2009, 10, 142. [Google Scholar] [CrossRef] [PubMed]
- Balage, M.; Larbaud, D.; Debras, E.; Hocquette, J.F.; Grizard, J. Acute hyperinsulinemia fails to change GLUT-4 content in crude membranes from goat skeletal muscles and adipose tissue. Comp. Biochem. Physiol. A Mol. Integr. Physiol. 1998, 120, 425–430. [Google Scholar] [CrossRef]
- Komatsu, T.; Itoh, F.; Kushibiki, S.; Hodate, K. Changes in gene expression of glucose transporters in lactating and nonlactating cows. J. Anim. Sci. 2005, 83, 557–564. [Google Scholar] [CrossRef] [PubMed]
- Kinoshita, A.; Locher, L.; Tienken, R.; Meyer, U.; Dänicke, S.; Rehage, J.; Huber, K. Associations between forkhead box O1 (FoxO1) expression and indicators of hepatic glucose production in transition dairy cows supplemented with dietary nicotinic acid. PLoS ONE 2016, 11, e0146670. [Google Scholar] [CrossRef] [PubMed]
- Fenwick, M.A.; Fitzpatrick, R.; Kenny, D.A.; Diskin, M.G.; Patton, J.; Murphy, J.J.; Wathes, D.C. Interrelationships between negative energy balance (NEB) and IGF regulation in liver of lactating dairy cows. Domest. Anim. Endocrinol. 2008, 34, 31–44. [Google Scholar] [CrossRef] [PubMed]
- Leturque, A.; Brot-Laroche, E.; Le Gall, M. GLUT2 mutations, translocation, and receptor function in diet sugar managing. Am. J. Physiol. Endocrinol. Metab. 2009, 296, E985-992. [Google Scholar] [CrossRef] [PubMed]
- Pausch, H.; Schwarzenbacher, H.; Burgstaller, J.; Flisikowski, K.; Wurmser, C.; Jansen, S.; Jung, S.; Schnieke, A.; Wittek, T.; Fries, R. Homozygous haplotype deficiency reveals deleterious mutations compromising reproductive and rearing success in cattle. BMC Genom. 2015, 16, 312. [Google Scholar] [CrossRef] [PubMed]
- Im, S.-S.; Kang, S.-Y.; Kim, S.-Y.; Kim, H.-I.; Kim, J.-W.; Kim, K.-S.; Ahn, Y.-H. Glucose-stimulated upregulation of GLUT2 gene is mediated by sterol response element-binding protein-1c in the hepatocytes. Diabetes 2005, 54, 1684–1691. [Google Scholar] [CrossRef] [PubMed]
- Van den Top, A.M.; Wensing, T.; Geelen, M.J.; Wentink, G.H.; van’t Klooster, A.T.; Beynen, A.C. Time trends of plasma lipids and enzymes synthesizing hepatic triacylglycerol during postpartum development of fatty liver in dairy cows. J. Dairy Sci. 1995, 78, 2208–2220. [Google Scholar] [CrossRef]
- Loor, J.J.; Dann, H.M.; Everts, R.E.; Oliveira, R.; Green, C.A.; Guretzky, N.A.J.; Rodriguez-Zas, S.L.; Lewin, H.A.; Drackley, J.K. Temporal gene expression profiling of liver from periparturient dairy cows reveals complex adaptive mechanisms in hepatic function. Physiol. Genom. 2005, 23, 217–226. [Google Scholar] [CrossRef] [PubMed]
- Drackley, J.K.; Andersen, J.B. Splanchnic metabolism of long-chain fatty acids in ruminants. In Ruminant Physiology: Digestion, Metabolism and Impact of Nutrition on Gene Expression, Immunology and Stress; Sejrsen, K., Hvelplund, T., Nielsen, M.O., Eds.; Wageningen Academic Publishers: Wageningen, The Netherlands, 2006; pp. 199–224. ISBN 978-90-76998-64-0. [Google Scholar]
- Graber, M.; Kohler, S.; Kaufmann, T.; Doherr, M.G.; Bruckmaier, R.M.; van Dorland, H.A. A field study on characteristics and diversity of gene expression in the liver of dairy cows during the transition period. J. Dairy Sci. 2010, 93, 5200–5215. [Google Scholar] [CrossRef] [PubMed]
- Murondoti, A.; Jorritsma, R.; Beynen, A.C.; Wensing, T.; Geelen, M.J.H. Unrestricted feed intake during the dry period impairs the postpartum oxidation and synthesis of fatty acids in the liver of dairy cows. J. Dairy Sci. 2004, 87, 672–679. [Google Scholar] [CrossRef]
- Li, X.; Li, Y.; Yang, W.; Xiao, C.; Fu, S.; Deng, Q.; Ding, H.; Wang, Z.; Liu, G.; Li, X. SREBP-1c overexpression induces triglycerides accumulation through increasing lipid synthesis and decreasing lipid oxidation and VLDL assembly in bovine hepatocytes. J. Steroid Biochem. Mol. Biol. 2014, 143, 174–182. [Google Scholar] [CrossRef] [PubMed]
- Greenfield, R.B.; Cecava, M.J.; Donkin, S.S. Changes in mRNA expression for gluconeogenic enzymes in liver of dairy cattle during the transition to lactation. J. Dairy Sci. 2000, 83, 1228–1236. [Google Scholar] [CrossRef]
- Bradford, B.J.; Allen, M.S. Phlorizin administration increases hepatic gluconeogenic enzyme mRNA abundance but not feed intake in late-lactation dairy cows. J. Nutr. 2005, 135, 2206–2211. [Google Scholar] [CrossRef] [PubMed]
- Hammon, H.M.; Philipona, C.; Zbinden, Y.; Blum, J.W.; Donkin, S.S. Effects of dexamethasone and growth hormone treatment on hepatic gluconeogenic enzymes in calves. J. Dairy Sci. 2005, 88, 2107–2116. [Google Scholar] [CrossRef]
- Loor, J.J.; Dann, H.M.; Guretzky, N.A.J.; Everts, R.E.; Oliveira, R.; Green, C.A.; Litherland, N.B.; Rodriguez-Zas, S.L.; Lewin, H.A.; Drackley, J.K. Plane of nutrition prepartum alters hepatic gene expression and function in dairy cows as assessed by longitudinal transcript and metabolic profiling. Physiol. Genom. 2006, 27, 29–41. [Google Scholar] [CrossRef] [PubMed]
- Hammon, H.M.; Sauter, S.N.; Reist, M.; Zbinden, Y.; Philipona, C.; Morel, C.; Blum, J.W. Dexamethasone and colostrum feeding affect hepatic gluconeogenic enzymes differently in neonatal calves. J. Anim. Sci. 2003, 81, 3095–3106. [Google Scholar] [CrossRef] [PubMed]
- Zammit, V.A. Role of insulin in hepatic fatty acid partitioning: Emerging concepts. Biochem. J. 1996, 314 Pt 1, 1–14. [Google Scholar] [CrossRef] [PubMed]
- Ingvartsen, K.L. Feeding- and management-related diseases in the transition cow: Physiological adaptations around calving and strategies to reduce feeding-related diseases. Anim. Feed Sci. Technol. 2006, 126, 175–213. [Google Scholar] [CrossRef]
- Farese, R.V.; Zechner, R.; Newgard, C.B.; Walther, T.C. The problem of establishing relationships between hepatic steatosis and hepatic insulin resistance. Cell Metab. 2012, 15, 570–573. [Google Scholar] [CrossRef] [PubMed]
- Prelusky, D.B.; Trenholm, H.L.; Lawrence, G.A.; Scott, P.M. Nontransmission of deoxynivalenol (vomitoxin) to milk following oral administration to dairy cows. J. Environ. Sci. Health Part B 1984, 19, 593–609. [Google Scholar] [CrossRef] [PubMed]
- Keese, C.; Meyer, U.; Valenta, H.; Schollenberger, M.; Starke, A.; Weber, I.-A.; Rehage, J.; Breves, G.; Dänicke, S. No carry over of unmetabolised deoxynivalenol in milk of dairy cows fed high concentrate proportions. Mol. Nutr. Food Res. 2008, 52, 1514–1529. [Google Scholar] [CrossRef] [PubMed]
- Zhou, H.-R.; Yan, D.; Pestka, J.J. Induction of cytokine gene expression in mice after repeated and subchronic oral exposure to vomitoxin (Deoxynivalenol): Differential toxin-induced hyporesponsiveness and recovery. Toxicol. Appl. Pharmacol. 1998, 151, 347–358. [Google Scholar] [CrossRef] [PubMed]
- Kushibiki, S.; Hodate, K.; Shingu, H.; Ueda, Y.; Shinoda, M.; Mori, Y.; Itoh, T.; Yokomizo, Y. Insulin resistance induced in dairy steers by tumor necrosis factor alpha is partially reversed by 2,4-thiazolidinedione. Domest. Anim. Endocrinol. 2001, 21, 25–37. [Google Scholar] [CrossRef]
- Schären, M.; Frahm, J.; Kersten, S.; Meyer, U.; Hummel, J.; Breves, G.; Dänicke, S. Interrelations between the rumen microbiota and production, behavioral, rumen-fermentation, metabolic, and immunological attributes of dairy cows. J. Dairy Sci. 2018. [Google Scholar] [CrossRef] [PubMed]
- Mishra, S.; Dwivedi, P.D.; Pandey, H.P.; Das, M. Role of oxidative stress in Deoxynivalenol induced toxicity. Food Chem. Toxicol. 2014, 72, 20–29. [Google Scholar] [CrossRef] [PubMed]
- Abuelo, A.; Hernández, J.; Benedito, J.L.; Castillo, C. Association of oxidative status and insulin sensitivity in periparturient dairy cattle: An observational study. J. Anim. Physiol. Anim. Nutr. 2016, 100, 279–286. [Google Scholar] [CrossRef] [PubMed]
- Neuvians, T.P.; Pfaffl, M.W.; Berisha, B.; Schams, D. The mRNA expression of insulin receptor isoforms (IR-A and IR-B) and IGFR-2 in the bovine corpus luteum during the estrous cycle, pregnancy, and induced luteolysis. Endocrine 2003, 22, 93–100. [Google Scholar] [CrossRef]
- Bionaz, M.; Loor, J.J. Identification of reference genes for quantitative real-time PCR in the bovine mammary gland during the lactation cycle. Physiol. Genom. 2007, 29, 312–319. [Google Scholar] [CrossRef] [PubMed]
- Untergasser, A.; Cutcutache, I.; Koressaar, T.; Ye, J.; Faircloth, B.C.; Remm, M.; Rozen, S.G. Primer3—New capabilities and interfaces. Nucleic Acids Res. 2012, 40, e115. [Google Scholar] [CrossRef] [PubMed]
- Zhang, Z.; Schwartz, S.; Wagner, L.; Miller, W. A greedy algorithm for aligning DNA sequences. J. Comput. Biol. J. Comput. Mol. Cell Biol. 2000, 7, 203–214. [Google Scholar] [CrossRef] [PubMed]
- Hellemans, J.; Mortier, G.; De Paepe, A.; Speleman, F.; Vandesompele, J. qBase relative quantification framework and software for management and automated analysis of real-time quantitative PCR data. Genome Biol. 2007, 8, R19. [Google Scholar] [CrossRef] [PubMed]
- Littell, R.C.; Henry, P.R.; Ammerman, C.B. Statistical analysis of repeated measures data using SAS procedures. J. Anim. Sci. 1998, 76, 1216–1231. [Google Scholar] [CrossRef] [PubMed]
- Holm, S. A Simple Sequentially Rejective Multiple Test Procedure. Scand. J. Stat. 1979, 6, 65–70. [Google Scholar] [CrossRef]
- R Development Core Team. R: A Language and Environment for Statistical Computing; R Foundation for Statistical Computing: Vienna, Austria, 2016. [Google Scholar]
Period 1 | Period 2 | ||||||||||||||||
---|---|---|---|---|---|---|---|---|---|---|---|---|---|---|---|---|---|
Group (n) 2 | p-Values of Fixed Effects | Group (n) 2 | p-Values of Fixed Effects | ||||||||||||||
CON (14) | MYC (13) | SEM | W | M | W × M | CON30 (7) | CON60 (7) | MYC30 (6) | MYC60 (7) | SEM | W | M | W × M | C | W × C | M × C × W | |
Glucose (mmol/L) | 3.53 | 3.43 | 0.06 | 0.013 | 0.19 | 0.35 | 3.95 | 3.96 | 3.69 | 3.89 | 0.10 | <0.001 | 0.12 | 0.58 | 0.28 | 0.79 | 0.35 |
NEFA 1 (log10, µmol/L) | 2.26 | 2.25 | 0.02 | 0.002 | 0.80 | 0.87 | 2.33 a | 2.11 b | 2.21 c | 2.14 b,c | 0.03 | 0.23 | 0.21 | 0.27 | <0.001 | 0.035 | 0.30 |
BHB 1 (log10, µmol/L) | 2.70 | 2.72 | 0.03 | 0.061 | 0.60 | 0.96 | 2.54 | 2.50 | 2.67 | 2.49 | 0.05 | <0.001 | 0.23 | 0.019 | 0.031 | 0.40 | 0.001 |
Insulin 1 (log10, µU/mL) | 0.57 | 0.59 | 0.03 | <0.001 | 0.66 | 0.91 | 0.43 | 0.72 | 0.67 | 0.81 | 0.06 | 0.017 | 0.016 | 0.45 | 0.002 | 0.24 | 0.71 |
RQUICKI | 0.65 | 0.64 | 0.02 | 0.005 | 0.62 | 0.55 | 0.66 | 0.61 | 0.59 | 0.57 | 0.02 | <0.001 | 0.048 | 0.60 | 0.17 | 0.97 | 0.36 |
Gene | Sampling Week | Group (n = 5/Group) 1 | p-Values of Fixed Effects 2 | |||||||||
---|---|---|---|---|---|---|---|---|---|---|---|---|
CON30 | CON60 | MYC30 | MYC60 | SEM | W | M | W × M | C | W × C | W × C × M | ||
SLC2A1 | 0 | 5.98 | 10.24 | 7.70 | 9.08 | 3.26 | <0.001 | 0.013 | 0.16 | 0.72 | 0.64 | 0.21 |
15 | 4.37 | 6.87 | 18.69 | 9.32 | 2.09 | |||||||
27 | 2.55 | 4.19 | 5.43 | 4.05 | 1.11 | |||||||
SLC2A4 | 0 | 4.67 | 3.67 | 5.29 | 4.38 | 0.92 | <0.001 | 0.36 | 0.30 | 0.24 | 0.55 | 0.007 |
15 | 8.95 | 6.69 | 6.69 | 6.72 | 0.92 | |||||||
27 | 3.82 ab | 6.99 b | 5.74 ab | 2.93 a | 0.92 | |||||||
IRA | 0 | 4.36 | 4.26 | 4.36 | 3.86 | 0.71 | 0.16 | 0.32 | 0.002 | 0.10 | 0.11 | 0.081 |
15 | 3.81 | 4.29 | 5.10 | 5.65 | 0.69 | |||||||
27 | 3.04 | 6.88 | 2.66 | 2.46 | 0.69 | |||||||
IRB | 0 | 4.55 | 4.88 | 5.11 | 4.15 | 0.71 | <0.001 | 0.039 | 0.001 | 0.32 | 0.11 | 0.090 |
15 | 4.74 | 5.95 | 6.61 | 5.20 | 0.69 | |||||||
27 | 3.06 | 7.39 | 2.62 | 2.21 | 0.69 |
Gene | Sampling Week | Group (n = 5/Group) 1 | p-Values of Fixed Effects 2 | |||||||||
---|---|---|---|---|---|---|---|---|---|---|---|---|
CON30 | CON60 | MYC30 | MYC60 | SEM | W | M | W × M | C | W × C | W × C × M | ||
SLC2A1 | 0 | 3.39 | 3.29 | 4.67 | 4.72 | 0.58 | 0.25 | <0.001 | 0.83 | 0.43 | 0.47 | 0.80 |
15 | 3.56 | 4.41 | 4.82 | 5.7 | 0.58 | |||||||
27 | 2.95 | 3.35 | 5.12 | 4.67 | 0.58 | |||||||
SLC2A2 | 0 | 4.25 | 5.47 | 3.95 | 5.61 | 1.32 | 0.005 | 0.038 | 0.009 | 0.58 | 0.39 | 0.52 |
15 | 7.16 | 8.03 | 6.63 | 7.70 | 1.32 | |||||||
27 | 11.83 | 8.99 | 4.56 | 5.54 | 1.32 | |||||||
IRA | 0 | 4.47 | 4.78 | 3.88 | 3.82 | 0.62 | 0.15 | 0.55 | 0.21 | 0.22 | 0.40 | 0.73 |
15 | 4.25 | 2.69 | 4.44 | 3.87 | 0.62 | |||||||
27 | 3.86 | 3.43 | 3.28 | 2.91 | 0.62 | |||||||
IRB | 0 | 2.19 | 3.08 | 2.65 | 2.96 | 0.35 | 0.52 | 0.58 | 0.56 | 0.68 | 0.20 | 0.34 |
15 | 3.17 | 2.73 | 2.43 | 2.72 | 0.35 | |||||||
27 | 3.06 | 3.05 | 3.18 | 2.66 | 0.35 |
Gene | Sampling Week | Group (n = 5/Group) 1 | p-Values of Fixed Effects 2 | ||||||||||
---|---|---|---|---|---|---|---|---|---|---|---|---|---|
CON30 | CON60 | MYC30 | MYC60 | SEM | W | M | W × M | C | W × C | W × C × M | |||
G6Pase | 0 | 6.68 | 6.48 | 5.55 | 7.13 | 1.14 | <0.001 | 0.60 | 0.051 | 0.41 | 0.023 | 0.89 | |
15 | 6.94 | 5.66 | 8.45 | 10.17 | 1.14 | ||||||||
27 | 11.91 | 8.65 | 9.59 | 7.62 | 1.14 | ||||||||
FBP1 | 0 | 5.67 | 6.65 | 4.26 | 5.83 | 1.46 | <0.001 | 0.027 | 0.13 | 0.53 | 0.23 | 0.40 | |
15 | 9.07 | 6.49 | 6.69 | 8.20 | 1.46 | ||||||||
27 | 15.27 | 9.83 | 7.79 | 8.56 | 1.46 | ||||||||
PC | 0 | 24.21 | 32.37 | 35.87 | 32.11 | 0.67 | <0.001 | 0.35 | 0.021 | 0.61 | 0.55 | 0.35 | |
15 | 19.65 | 14.51 | 15.82 | 12.10 | 3.62 | ||||||||
27 | 21.98 | 14.70 | 5.13 | 5.88 | 3.03 | ||||||||
PCK1 | 0 | 12.80 | 14.75 | 14.31 | 17.11 | 2.35 | <0.001 | 0.69 | 0.61 | 0.073 | 0.012 | 0.84 | |
15 | 18.80 | 10.37 | 16.71 | 10.40 | 2.35 | ||||||||
27 | 6.86 | 4.06 | 8.53 | 4.23 | 2.35 | ||||||||
PCK2 | 0 | 3.42 | 3.94 | 4.78 | 4.77 | 0.59 | 0.005 | 0.69 | 0.017 | 0.12 | 0.25 | 0.84 | |
15 | 4.98 | 4.04 | 5.95 | 5.31 | 0.59 | ||||||||
27 | 6.62 | 5.81 | 5.85 | 4.44 | 0.59 | ||||||||
PCCA | 0 | 2.57 | 3.42 | 2.73 | 3.43 | 0.67 | <0.001 | 0.69 | 0.28 | 0.46 | 0.093 | 0.43 | |
15 | 4.42 | 3.63 | 4.90 | 4.96 | 0.67 | ||||||||
27 | 6.07 | 5.42 | 6.32 | 4.23 | 0.67 | ||||||||
PYGL | 0 | 6.77 | 6.84 | 5.70 | 8.54 | 1.25 | 0.20 | 0.88 | 0.70 | 0.50 | 0.37 | 0.35 | |
15 | 16.26 | 5.25 | 9.83 | 12.92 | 4.37 | ||||||||
27 | 11.67 | 5.57 | 5.35 | 8.21 | 2.28 |
Gene | Sampling Week | Group (n = 5/Group) 1 | p-Values of Fixed Effects 2 | |||||||||
---|---|---|---|---|---|---|---|---|---|---|---|---|
CON30 | CON60 | MYC30 | MYC60 | SEM | W | M | W × M | C | W × C | W × C × M | ||
ACSL1 | 0 | 2.41 | 3.57 | 1.96 | 2.10 | 0.51 | 0.83 | 0.049 | 0.18 | 0.70 | 0.45 | 0.15 |
15 | 3.04 | 2.20 | 2.58 | 3.02 | 0.51 | |||||||
27 | 3.67 | 2.74 | 1.82 | 2.55 | 0.51 | |||||||
CPT1A | 0 | 4.86 | 5.67 | 4.82 | 6.09 | 0.76 | 0.096 | 0.37 | 0.032 | 0.83 | 0.031 | 0.60 |
15 | 4.37 | 4.01 | 4.43 | 5.27 | 0.72 | |||||||
27 | 7.86 | 4.98 | 3.89 | 3.25 | 1.26 | |||||||
HMGS2 | 0 | 5.09 | 6.12 | 5.30 | 7.10 | 1.39 | <0.001 | 0.13 | 0.56 | 0.44 | 0.099 | 0.52 |
15 | 8.60 | 6.45 | 10.21 | 8.00 | 1.39 | |||||||
27 | 10.11 | 10.06 | 14.11 | 11.02 | 1.39 | |||||||
HMGL | 0 | 26.01 | 28.92 | 24.11 | 30.73 | 0.50 | <0.001 | 0.41 | 0.071 | 0.11 | 0.074 | 0.38 |
15 | 44.76 | 25.48 | 45.21 | 48.37 | 0.50 | |||||||
27 | 51.03 | 36.67 | 42.93 | 35.95 | 0.50 | |||||||
BDH2 | 0 | 3.11 | 2.78 | 4.70 | 3.18 | 0.75 | <0.001 | 0.50 | 0.35 | 0.013 | 0.15 | 0.50 |
15 | 4.74 | 3.31 | 5.18 | 3.94 | 0.75 | |||||||
27 | 7.23 | 4.14 | 6.44 | 4.30 | 0.75 | |||||||
ACCA | 0 | 3.89 | 5.05 | 5.79 | 3.78 | 1.63 | 0.001 | 0.35 | <0.001 | 0.24 | 0.91 | 0.091 |
15 | 9.42 | 4.28 | 9.53 | 9.68 | 1.63 | |||||||
27 | 12.53 | 11.56 | 6.45 | 5.07 | 1.63 | |||||||
FASN | 0 | 3.72 | 2.09 | 4.44 | 2.43 | 0.77 | 0.002 | 0.74 | 0.19 | 0.49 | 0.40 | 0.65 |
15 | 6.65 | 5.42 | 6.64 | 8.19 | 1.79 | |||||||
27 | 6.83 | 6.09 | 3.50 | 3.77 | 1.61 | |||||||
GPAM | 0 | 5.92 | 7.81 | 5.43 | 6.74 | 1.14 | 0.026 | 0.51 | 0.002 | 0.047 | 0.91 | 0.26 |
15 | 7.40 | 6.06 | 7.94 | 11.18 | 1.14 | |||||||
27 | 9.72 | 10.92 | 6.05 | 7.87 | 1.14 | |||||||
DGAT1 | 0 | 1.48 | 1.90 | 2.12 | 1.67 | 0.15 | 0.001 | 0.64 | 0.14 | 0.36 | 0.48 | 0.20 |
15 | 2.09 | 1.91 | 2.42 | 2.19 | 0.30 | |||||||
27 | 2.20 | 2.23 | 2.19 | 1.68 | 0.16 |
Period 1 | Period 2 | |||||
---|---|---|---|---|---|---|
CON | MYC | CON30 | CON60 | MYC30 | MYC60 | |
Ingredients (%) | ||||||
Triticale | 25 | 10.5 | 15 | 30 | 6.3 | 12.6 |
Fusarium-contaminated triticale | 0 | 14.5 | 0 | 0 | 8.7 | 17.4 |
Soybean meal | 13.35 | 13.35 | 8.01 | 16.02 | 8.01 | 16.02 |
Maize | 10.35 | 10.35 | 6.21 | 12.42 | 6.21 | 12.42 |
Mineral feed | 0.7 | 0.7 | 0.42 | 0.84 | 0.42 | 0.84 |
Calcium carbonate | 0.6 | 0.6 | 0.36 | 0.72 | 0.36 | 0.72 |
Maize silage | 25 | 25 | 35 | 20 | 35 | 20 |
Grass silage | 25 | 25 | 35 | 20 | 35 | 20 |
Dry matter [g/kg] | 452 | 465 | 386 | 456 | 394 | 475 |
Nutrients [g/kg DM] | ||||||
Crude ash | 6 | 61 | 68 | 61 | 69 | 66 |
Crude protein | 150 | 153 | 135 | 165 | 139 | 154 |
Crude fat | 25 | 26 | 28 | 26 | 28 | 27 |
Crude fiber | 143 | 143 | 203 | 142 | 196 | 128 |
Acid detergent fiber | 157 | 157 | 220 | 161 | 212 | 169 |
Neutral detergent fiber | 305 | 307 | 391 | 349 | 394 | 349 |
Metabolizable energy [MJ/kg DM] | 11.6 | 11.6 | 11.4 | 12.4 | 11.6 | 12.1 |
Net energy lactation [MJ/kg DM] | 7.1 | 7.1 | 7.0 | 7.8 | 7.1 | 7.5 |
Mycotoxins | ||||||
Deoxynivalenol [mg/kg DM] | 0.6 | 5.3 | 0.6 | 0.4 | 4.4 | 4.6 |
Zearalenone [µg/kg DM] | 63.1 | 112.7 | 35.0 | 24.4 | 73.8 | 72.5 |
Primer Sequences (5′-3′) 2 | Accession Number | Size (Base) 3 | Tm (°C) 4 | Tissue 5 | Linear Dynamic Range(ng RNA) 6 | E 7 | |
---|---|---|---|---|---|---|---|
SLC2A1 1 | F-AGATGATGCGGGAGAAGAAG | BC119940 | 238 | 89 | L | 6.25–100 | 1.86 (0.98) |
R-TCCACCACAAATAGCGACAC | M | 6.25–100 | 1.99 (0.95) | ||||
SLC2A4 | F-CCAACTGGACATGCAACTTCATC | BC114082 | 206 | 85 | M | 3.13–67.5 | 1.90 (0.99) |
R-CACTTCCTGCTCCAGAAGAGA | |||||||
FBP1 | F-CAACATCGACTGCCTTGTGT | BC102974 | 224 | 88 | L | 1.56–50 | 1.97 (0.99) |
R-CCCTGTCCACCAAAATGAAC | |||||||
PCK1 | F-AACTCGCTCCTTGGGAAGAA | BC112664 | 261 | 89 | L | 3.13–50 | 1.91 (0.99) |
R-CCGCAAGTTACCTTGTTGGT | |||||||
PCK2 | F-CATTGACGCCATCATCTTTG | BC102244 | 150 | 88 | L | 3.13–50 | 1.87 (0.99) |
R-TGCATGATGACCTTCCCTTT | |||||||
PC | F-CTATGTTGCCCACAGCTTCA | BC114135 | 244 | 90 | L | 3.13–50 | 1.93 (0.98) |
R-GATGTCCATGCCATTCTCCT | |||||||
CPT1A | F-CCTATTTTGGACACGGGAAA | XM_002699420.1 | 172 | 86.5 | L | 3.13–50 | 1.78 (0.99) |
R-TCAAACCACCTGTCGAAACA | |||||||
HMGS2 | F-GGCCTTTCACTCTCGATGAT | BC112666 | 170 | 85 | L | 1.56–50 | 1.93 (0.99) |
R-TTCCAGCTTTAGTCCCCTGA | |||||||
HMGL | F-CAGCTTCGTGTCTCCCAAAT | BC118276 | 227 | 87 | L | 3.13–50 | 1.87 (0.99) |
R-ACCGCTGCAAACTCTCATCT | |||||||
BDH2 | F-TATCCGGTGCAACTGTGTGT | BC102567 | 204 | 84 | L | 3.13–50 | 2.01 (0.98) |
R-ACAGGATTCCCCGTGATGTA | |||||||
ACCA | F-ATCCGACGCCTTACTTTCCT | AJ132890 | 193 | 82.5 | L | 3.13–50 | 2.05 (0.98) |
R-TTCTCATCCGGTTCAGCTCT | |||||||
FASN | F-AACACCTCGGTGCAGTTCAT | AY343889 | 196 | 88 | L | 3.13–50 | 1.94 (0.99) |
R-CTTTCTCTTTGCAGCCATCC | |||||||
GPAM | F-GCCAGATGGACGGAAAGATA | AY515690 | 182 | 83.5 | L | 3.13–50 | 2.00 (0.97) |
R-TCGTATTGGTGGACAGCGTA | |||||||
DGAT1 | F-CTCTGTGCCTGGTCATTGTG | BC118146 | 239 | 90 | L | 3.13–50 | 1.89 (0.99) |
R-CCGGTAGGAGAACAGCTTGA | |||||||
ACSL1 | F-GAACTACAGGCAACCCCAAA | BC119914 | 240 | 83 | L | 3.13–50 | 1.94 (0.99) |
R-GGGCCTTGAGATCATCCATA | |||||||
GUSB | F-ACCAGAAGCCGATGATTCAG | BC133415 | 160 | 84 | L | 3.13–75 | 1.96 (0.99) |
R-AGCTCGCCAACCACATATTC | M | 6.25–100 | 1.76 (0.96) |
© 2018 by the authors. Licensee MDPI, Basel, Switzerland. This article is an open access article distributed under the terms and conditions of the Creative Commons Attribution (CC BY) license (http://creativecommons.org/licenses/by/4.0/).
Share and Cite
Kinoshita, A.; Keese, C.; Meyer, U.; Starke, A.; Wrenzycki, C.; Dänicke, S.; Rehage, J. Chronic Effects of Fusarium Mycotoxins in Rations with or without Increased Concentrate Proportion on the Insulin Sensitivity in Lactating Dairy Cows. Toxins 2018, 10, 188. https://doi.org/10.3390/toxins10050188
Kinoshita A, Keese C, Meyer U, Starke A, Wrenzycki C, Dänicke S, Rehage J. Chronic Effects of Fusarium Mycotoxins in Rations with or without Increased Concentrate Proportion on the Insulin Sensitivity in Lactating Dairy Cows. Toxins. 2018; 10(5):188. https://doi.org/10.3390/toxins10050188
Chicago/Turabian StyleKinoshita, Asako, Christina Keese, Ulrich Meyer, Alexander Starke, Christine Wrenzycki, Sven Dänicke, and Jürgen Rehage. 2018. "Chronic Effects of Fusarium Mycotoxins in Rations with or without Increased Concentrate Proportion on the Insulin Sensitivity in Lactating Dairy Cows" Toxins 10, no. 5: 188. https://doi.org/10.3390/toxins10050188
APA StyleKinoshita, A., Keese, C., Meyer, U., Starke, A., Wrenzycki, C., Dänicke, S., & Rehage, J. (2018). Chronic Effects of Fusarium Mycotoxins in Rations with or without Increased Concentrate Proportion on the Insulin Sensitivity in Lactating Dairy Cows. Toxins, 10(5), 188. https://doi.org/10.3390/toxins10050188